What is it?
Indirect calorimetry is the science associated with analysing metabolic processes. While direct calorimetry is achieved through direct measurement of total body heat produced, such as via a thermally sealed chamber, indirect calorimetry measures respiratory gases, i.e. oxygen (O2) and carbon dioxide (CO2) that are influenced by metabolism to meet energy requirements (Figure 1). There are several different methods of indirect calorimetry for studying the metabolic systems which include expired gas analysis, double labelled water and nitrogen excretion. Generally, the most widely accepted method is expired gas analysis indirect calorimetry (EGAIC) which is frequently used to accurately study steady state metabolic conditions and exercise physiology. EGAIC can be performed in an open circuit system with a metabolic cart or in a closed circuit system with a respiration chamber.
Fundamental principles
Macronutrients and human metabolism
During metabolism, basic macronutrients in food (carbohydrates, fats and proteins) are fuel for energy. As such, macronutrients are catabolised for energy in the presence of inspired and converted into , water and heat (Figure 1). Depending on the composition of macronutrients, the volume of oxygen consumed (VO2) and carbon dioxide produced (VCO2) and the amount of energy liberated varies (Table 1). Likewise, catabolism of different macronutrients yield different amounts of energy relative to oxygen consumed, termed the caloric equivalent for oxygen. Therefore by determining the VO2 and VCO2, energy expenditure can be quantified.
TABLE 1: Comparison of oxygen consumed, carbon dioxide released, respiratory quotient, energy generation during oxidation of 3 main macronutrients
Macronutrient | consumed1 | produced1 | RQ1 | Energy (Atwater factors)3 |
(g) | (L/g) | (L/g) | (kCal/g) | |
Carbohydrate | 0.829 | 0.829 | 1.00 | 4 |
Fat | 2.019 | 1.427 | 0.71 | 9 |
Protein | 0.966 | 0.774 | 0.80 | 4 |
What are the outcomes?
Multiple outcome parameters can be derived from indirect calorimetry measurements depending on user needs and applications. These include nutritional parameters (e.g. carbohydrate and fat utilization), metabolic rate (e.g. total energy expenditure) and aerobic fitness (e.g. ventilatory thresholds, maximum oxygen consumption). The most common outcome parameters are the respiratory exchange ratio (RER), energy expenditure (EE) and maximum oxygen consumption (VO2max).
Respiratory exchange ratio (RER)
The respiratory energy ratio (RER) or respiratory quotient (RQ), is the ratio between VO2 and VCO2. Under resting steady state conditions, RQ is used to represent net substrate (carbohydrates, fat and protein) oxidation by the body based on respiratory gas exchanges. Under most circumstances, RQ is between 0.7 to 1.0. RQ values obtained from clinical patients outside this range should trigger further questioning. A larger RQ value could reflect an increase in VCO2 caused by excess exhalation of carbon dioxide as a result of underlying compensatory mechanisms such as metabolic acidosis or hyperventilation. On the other hand during intense exercise, RER values can be larger than 1.2 due to generation of carbon dioxide from anaerobic energy metabolism, in excess of that from aerobic metabolism.
Energy Expenditure (EE)
Energy expenditure is often used to calculate energy requirements. The total energy expenditure (TEE) is defined as the energy used by the human body for daily function and can be divided into three main components (Figure 2): the basal metabolic rate (BMR), accounts for approximately 60% to 75% of our total energy output, the thermic effect of food (TEF; also known as diet-induced thermogenesis), and the energy cost of physical activity. Energy cost of physical activity is the most variable component of TEE, which accounts for energy consumed in muscular work during spontaneous and voluntary exercise and varies depending on individual physical activity levels. TEF refers to the energy cost in relation to food consumption, i.e. to digest, absorb, assimilate, and store nutrients, and thus is dependent on the amount and the type of nutrients consumed. Key determinants of the energy cost of the components are illustrated in Figure 2.
BMR can be derived accurately using indirect calorimetry by measuring VO2 and VCO2 under standard conditions (being awake in the supine position, ten to 12 hours after a meal, following eight hours of physical rest and no strenuous exercise in the preceding day, and being in a state of mental relaxation and an ambient environmental temperature that does not evoke shivering or sweating), on the assumption that the volume of oxygen consumed is used to catabolise macronutrients and all the carbon dioxide produced is recovered. Most indirect calorimetry systems use the abbreviated Weir equation to calculate EE, expressed as:
EE (kCal/day) = (3.94 x VO2) + (1.1 x VCO2) x 1440
Although there are predictive equations for calculating BMR or TEE based on measurements that are easier to obtain, such as body weight and/or height, these estimations are often inaccurate due to inter-individual diversity and various factors that influence TEE. Furthermore, these equations are developed based on energy expenditure of healthy individuals.
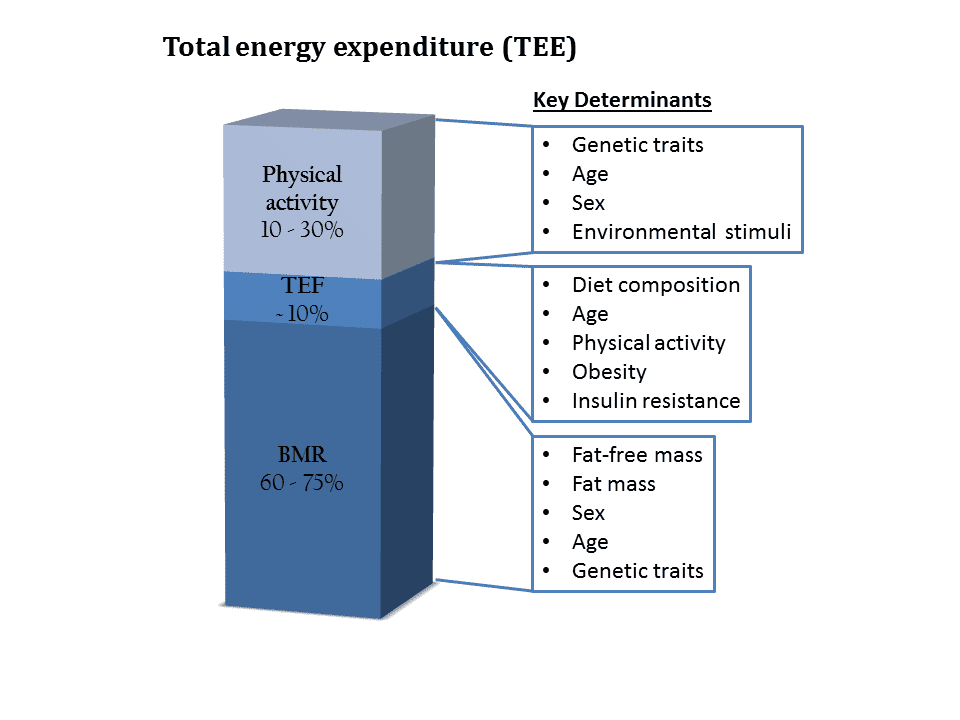
Maximum rate of oxygen consumption (VO2max)
The maximum rate of oxygen consumption (VO2max) or aerobic capacity is defined as the highest rate at which oxygen can be taken up, transported and utilized by the body for producing energy. Determination of VO2max is performed by measuring oxygen uptake (VO2) using expired gas indirect calorimetry during incremental exercise to volitional exhaustion. VO2max has numerous applications in exercise physiology and clinical fields such as cardiology and pulmonology.
Main Applications
Indirect calorimetry is a reliable and accurate tool for studies of energy expenditure. Quantifying energy expenditure has multiple applications and is used most often to determine metabolic rate, assess physical fitness and nutritional needs and the efficacy of treatment or prevention programs.
The main applications include:
- Sports and performance testing
- Research and clinical trials
- Nutrition
- Metabolic Syndrome
- Economy and efficiency
- Hospital Care
Technologically advanced indirect calorimetry systems such as the Omnical and room calorimeter developed by Maastricht Instruments BV, provide a user-friendly, accurate and reliable option to support research and clinical applications.
Contact us to find out more about indirect calorimetry and our instruments.
References
- Jequier E, Acheson K, Schutz Y. Assessment of energy expenditure and fuel utilization in man. Annual review of nutrition. 1987 Jul;7(1):187-208.
- Joseph Feher, 8.6 – Energy Balance and Regulation of Food Intake, In Quantitative Human Physiology, Academic Press, Boston, 2012, Pages 744-756.
- De V, Weir JB. New methods for calculating metabolic rate with special reference to protein metabolism. J Physiol 1949; 109: 1–9.
- von Loeffelholz C. The Role of Non-exercise Activity Thermogenesis in Human Obesity. [Updated 2014 Jun 5]. In: De Groot LJ, Chrousos G, Dungan K, et al., editors. Endotext [Internet]. South Dartmouth (MA): MDText.com, Inc.; 2000-. Available from: https://www.ncbi.nlm.nih.gov/books/NBK279077/
- Lam YY, Ravussin E. Analysis of energy metabolism in humans: A review of methodologies. Molecular Metabolism. 2016;5(11):1057-1071.
- Boone, Tommy. Introduction to exercise physiology. Jones & Bartlett Publishers, 2013.